“The real challenge is getting quantum sensors out of the lab and into products that we can make on an industrial scale.”
The second quantum revolution has the potential to upend the world of sensors as we know it… provided that practical industrial applications can be found for these new technologies... Bertrand Demotes, VP Key Technical Domain Hardware, outlines how this will be achievable.
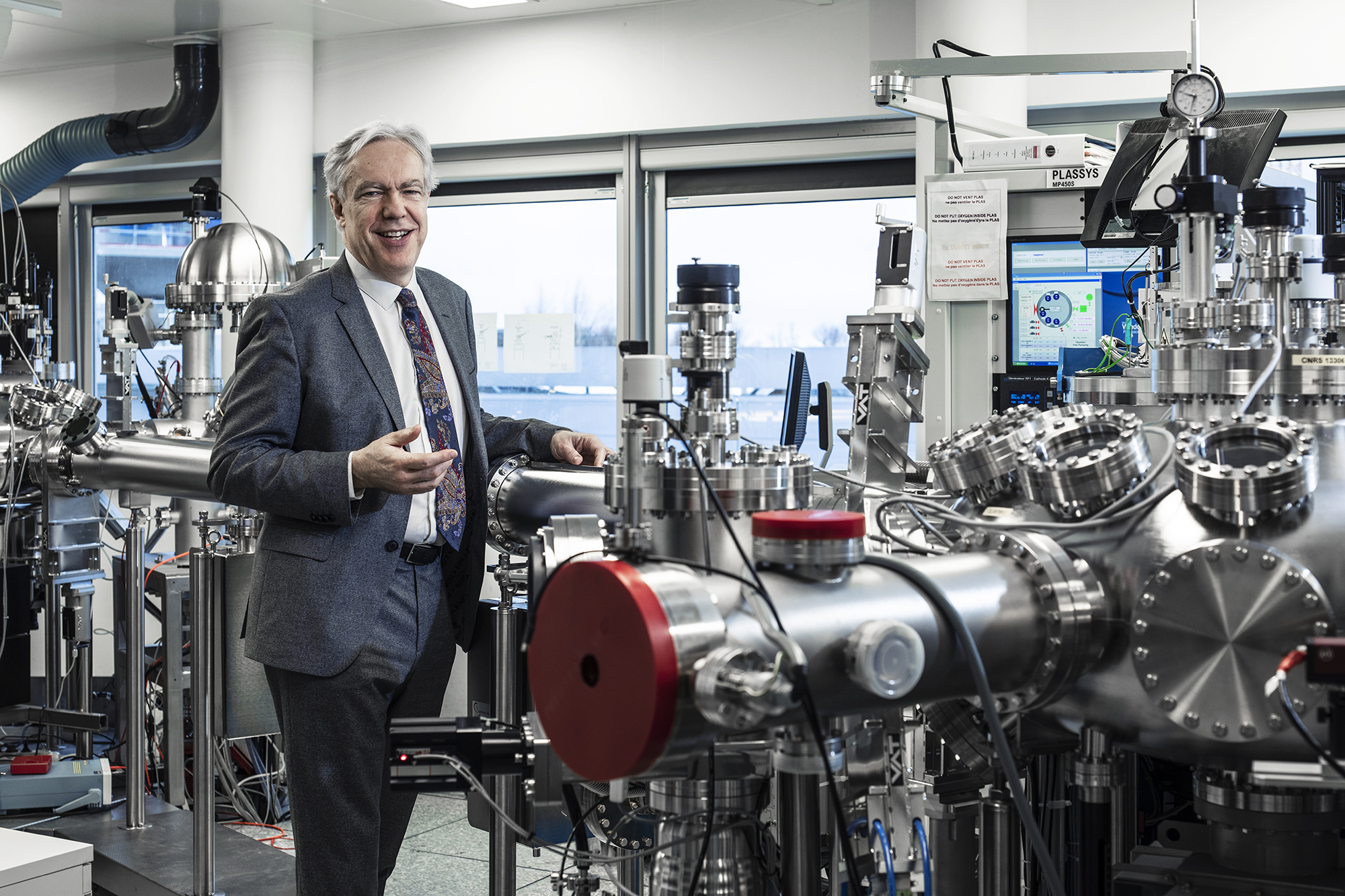
Alain Aspect, winner of the 2022 Nobel Prize in Physics for his pioneering work on photon entanglement, referred to the “second quantum revolution” when he described the vast potential for innovation unlocked by recent advances in quantum physics. Is this new paradigm also about to revolutionise the world of sensors as we know it?
Quantum physics dates back over a hundred years, and it’s important to understand that many objects that we use today in the field of metrology* – such as transistors, chips for computers and mobile phones, lasers, hard disks, and global positioning devices – are directly descended from the discoveries made in the wake of the first quantum revolution. In fact, all of the electronic and optronic devices that power our information society rely on the disruptive technologies unleashed by advances in quantum physics. The applications that arose out of that first wave, however, only apply these new laws to objects that are “large” in microscopic terms, because they make use of large quantities of atoms or particles. A laser, for example, emits huge volumes of photons, while the operation of a transistor depends on applying a current to enable clouds of electrons to flow in one direction or another. About forty years ago – Alain Aspect’s work on quantum entanglement goes right back to 1986 – scientists realised that it was possible to manipulate a small number of particles, or even single particles, for example by trapping a single electron or a single ion, or by controlling the emission of pairs of photons. This ability to seek out the individual effects of matter, rather than relying only on mean (or average) effects, theoretically opens the door to a whole new world of innovations which could exploit other aspects of quantum physics. It offers huge potential in my area – sensors – provided that all this scientific learning can be turned into concrete applications we can produce on an industrial scale.
So as things stand at the moment, innovation in quantum sensors is more a matter for engineers than researchers?
In a field as demanding as quantum physics, we obviously do need to rely on the fundamental science. At Thales, we devote a great deal of energy to fundamental research. In almost twenty years of existence, our laboratory at Palaiseau, for example, has built a global reputation for its work on future quantum sensors. It's a crucial part of the equation, and it enables our teams to benefit from the huge amount of academic research that has been carried out on the topic and pass it on to the engineers responsible for developing real products. Innovation, whether in the field of sensors or any other area of industry, is less about making major scientific breakthroughs and more about finding practical applications and developing viable products for our markets. In the quantum world, identifying use cases is, in many ways, almost as complicated as the scientific research itself.
Which technologies are the most likely to find viable use cases first?
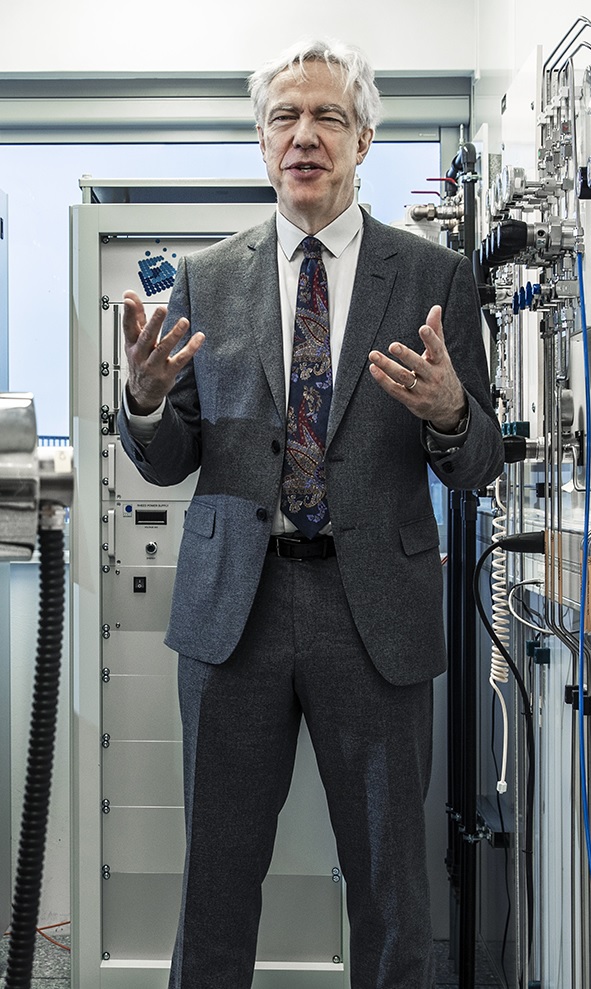
We have identified a dozen or so different technologies with the potential to open up new opportunities. Not all of them are at the same level of maturity, but three technologies that we are keeping a close eye on stand out from the rest. The first relates to what are known as NV (nitrogen-vacancy) centres in diamonds. In simple terms, this technology involves making use of a defect in the atomic lattice of the diamond to trap systems of particles within the crystalline structure itself. This mechanism, which is by nature very stable, potentially paves the way for the development of new types of sensors, for example to measure magnetic fields on a nanometric scale. This is one of the aims of the ASTERIQs pilot project led by Thales.
Another area we are interested in is cold atom technology, which involves isolating particles in a vacuum cell** so they can be cooled down and held in place using pairs of lasers. This enables us to create what we call a Bose-Einstein condensate, which behaves as a single quantum object, from which we could create the atomic-scale equivalent of a laser inertial navigation system***. The many potential applications of this technology include navigation systems which are not dependent on global positioning, and which could be used in space exploration, or even for guiding fleets of driverless cars. But first there is an issue of size that needs to be overcome: how do we scale down the experimental cold atom laser system, which currently needs to be housed in a chamber measuring around 7 cubic metres, to fit in a package of just a few cubic centimetres that could be incorporated into a device?
Our third priority sector is SQIFs (Superconducting Quantum Interference Filters). This technology is more mature than the others, and could be the first to find concrete applications.
How do SQIFs work, and what could they be used for?
SQIFS are interference filters relying on the laws of quantum physics that govern superconductivity. They use a type of magnetometer known as a SQUID (Superconducting Quantum Interference Device), which has been the focus of intensive research and experimentation for several decades. SQUIDs rely on a phenomenon known as the Josephson Effect, and are capable of detecting extremely weak magnetic fields when cooled to temperatures close to absolute zero. Analysing the measurements provided by these tiny cells is a long and difficult process, but the signal can be amplified and is less ambiguous when several SQUIDs are arranged in series. At Thales, we have developed a method for combining a thousand SQUIDs to produce a SQIF, which offers a combination of benefits such as very high sensitivity to magnetic waves, compact design and wide frequency coverage. SQIFs are therefore a very good alternative to conventional antennas, particularly at long wavelengths, where larger-sized devices are required. The many potential applications of SQIF antennas include long-range communication systems for submarines, which use very long wavelengths. In this field in particular, these new quantum sensors are extremely promising.
What are the major challenges that need to be overcome to allow these second-generation quantum sensors to break through into our day-to-day lives?
Understanding the technology behind quantum sensors is clearly a crucial first step and a vital prerequisite for any project. Important as it is, however, this stage only accounts for a small proportion of our work on next-generation sensors. Once this foundation is in place, we have to build everything else. These devices start life on laboratory workbenches, operating under experimental conditions, but the big challenge is to convert them into industrial products that we can manufacture at scale for specific uses. It means getting engineers and researchers from a wide range of specialisms and scientific disciplines to work together – and Thales has a great deal of experience in doing just that. Indeed, one of our distinctive strengths is the ability to combine the capabilities of experts from different fields to move a project forward. Our production facility at Châtellerault, for example, with its world-class capabilities in gyrolaser manufacture, owes its success to the ability to get experts in vacuum technology, electronics, diodes, lasers and other fields to work together. We aim to recreate this type of ecosystem to help us develop the first concrete applications based on quantum sensors.
We already have many of the critical skills right here at Thales. In cooling systems, for example – key to the development of SQIFs – we can draw on over 25 years of experience in the development and manufacture of very similar systems that we use for our infrared sensors.
For young graduates and researchers, joining Thales is a unique opportunity to work in a multi-disciplinary environment and challenge everything they took for granted. It's a chance to ask endless questions and learn how to think about things differently. In the promising new field of quantum sensors, history is in the making – and we’re writing the next chapters. And that’s what’s make it so fascinating!
*The science of measurement, which, by definition, makes use of a range of different types of sensors.
**A confined space from which air and other gases have been removed using a vacuum pump.
***A type of navigation system using gyrolaser (laser gyroscope) technology to determine the orientation of a moving object in a 3D space by measuring its speed and position.